Prevention of Surgical Site Infections and Biofilms: Pharmacokinetics of Subcutaneous Cefazolin and Metronidazole in a Tumescent Lidocaine Solution
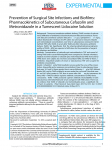
BACKGROUND: Tumescent anesthesia antibiotic delivery (TAAD) consists of subcutaneous infltration of antibiotic(s) dissolved tumescent lidocaine anesthesia. Tumescent lidocaine anesthesia contains lidocaine (≤ 1g/L), epinephrine (≤ 1mg/L), sodium bicarbonate (10 mEq/L) in 0.9% saline. Our aim was to measure cefazolin and metronidazole concentrations over time in subcutaneous tumescent interstitial fluid (TISF) after TAAD, in serum after TAAD and after intravenous antibiotic delivery (IVAD). We hypothesize that the pharmacokinetic/pharmacodynamic profles of TAAD + IVAD are superior to IVAD alone for the prevention of surgical
site infections and bioflms.
METHODS: Concentrations of cefazolin and metronidazole in TISF and serum following TAAD and in serum following IVAD were compared in 5 female volunteers. Subjects received cefazolin or cefazolin plus metronidazole by IVAD alone and by TAAD alone. One subject also received concomitant IVAD and TAAD of these 2 antibiotics. Sequential samples of serum or subcutaneous TISF were assayed for antibiotic concentration.
RESULTS: Cefazolin (1g) by TAAD resulted in an area under the curve of the concentration–time profle and a maximum concentration (Cmax) in subcutaneous tissue that were 16.5 and 5.6 times greater than in serum following 1g by IVAD. Metronidazole (500mg) by TAAD resulted in an area under the curve and Cmax that were 8.1 and 24.7 times greater in TISF, than in serum after 500mg by intravenous delivery. IVAD + TAAD resulted in superior antibiotic concentrations to IVAD alone.
CONCLUSIONS: Conclusions: TAAD + IVAD produced superior antibiotic bioavailability in both subcutaneous interstitial fluid and serum compared with IVAD alone. There was no evidence that TAAD of cefazolin and metronidazole poses a signifcant risk of harm to patients. (Plast Reconstr Surg Glob Open 2017;5:e1351; doi: 10.1097/GOX.0000000000001351; Published online 30 May 2017.)
INTRODUCTION
Surgical site infection (SSI) and bacterial drug resistance remain major problems.1–3 Intravenous antibiotic delivery (IVAD) may not reliably achieve adequate subcutaneous antibiotic concentrations.4-ics, dissolved in saline, before incision reduce the risk of SSI.9–14 TAAD is a novel mode of drug delivery that delays systemic drug absorption and prolongs local subcutaneous drug effects.
This research was an exploratory phase 1 pharmacokinetic clinical trial comparing the subcutaneous and systemic bioavailability of antibiotics following TAAD or IVAD. After TAAD, subcutaneous interstitial fluid is designated tumescent interstitial fluid (TISF).
The principal aim of this research was to measure concentrations of cefazolin and metronidazole over time in subcutaneous tissue and serum following TAAD and in serum following intravenous (IV) delivery. We hypothesize that, at equal doses, TAAD provides uniformly greater subcutaneous antibiotic concentrations, area under the curve (AUC∞), maximum concentrations (Cmax), and T > minimum inhibitory concentration (MIC; duration of time that drug concentration exceeds MIC) compared with IV delivery.
A secondary research aim was to determine the correlation between the antibiotic concentration (mg/L) in a TAAD solution and the resulting antibiotic concentration (mg/L) in TISF immediately after tumescent delivery. We hypothesized that these 2 concentrations are highly correlated and nearly equal.
Another secondary research aim was to observe the concentration–time profles of cefazolin and metronidazole in serum and TISF after subcutaneous tumescent infltration. We hypothesize that, at equal antibiotic doses and equal concentration in TAAD solution, concentration–time profles of cefazolin and metronidazole in TISF are virtually identical. Further, we hypothesize that systemic antibiotic absorption following TAAD has a concentration–time profle in serum that resembles a slow constant IV infusion.
Adding cefazolin to a TAAD solutions involved withdrawing 10mL from the TLA solution and injecting it into a vial of cefazolin powder then injecting the solubilized cefazolin into the bag of TLA solution. Adding metronidazole to a TAAD solution involved transferring 500mg in 100mL into 1,110mL of a TLA solution.
Subjects 1, 2, and 3 received cefazolin once by IV infusion and then twice by tumescent infltration. Subject 4 received cefazolin and metronidazole dissolved in a single IV bag on 1 occasion by IVAD and by TAAD into abdomen on another occasion.
Subject 5 received concurrent cefazolin and metronidazole, once by IVAD, once by TAAD, and once by concomitant IVAD + TAAD. Procedures for individuals were at least 7 days apart to assure complete antibiotic clearance before a subsequent study.
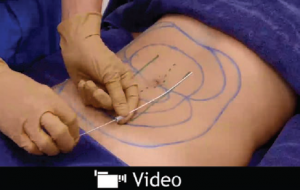
Video Graphic 1. See video, Supplemental Digital Content 10, which demonstrates the preferred technique for subcutaneous infiltration of TAAD solution using a multiholed plastic subcutaneous catheter, HK SubQKath. This video is available in the related Videos section of the Full-Text article on PrSGlobalOpen.com or available at http://links.lww.com/PRSGO/A443.
Subcutaneous tumescent infltration was accomplished using a peristaltic pump, infltration tubing, and Monty infltration cannulas (HKSurgical.Com, San Clemente, Calif.). All patients were fully awake and received no parenteral sedation (see videos, Supplemental Digital Content 10, which demonstrates the preferred technique for subcutaneous infltration of TAAD solution using a multiholed plastic subcutaneous catheter, HK SubQKath, http://links.lww.com/PRSGO/A443 and Supplemental Digital Content 11, which demonstrates the technique for painless subcutaneous infltration of large volumes of TLA using multiholed stainless steel cannulas, http://links.lww.com/PRSGO/A444).
IV antibiotic infusion was accomplished over 5 minutes via an antecubital vein. Serum samples were obtained via an indwelling IV catheter in a contralateral antecubital vein. After IVAD, only serum antibiotics concentrations were measured. After TAAD, serum and TISF concentrations were measured.
Blood sampling technique frst removed 2mL of blood, which was discarded; then 10mL of blood was obtained in a second syringe for drug assay. Finally, the IV catheter was flushed with 2mL saline and then heparin (5 units/0.5mL). Beginning at time t = 0 immediately after antibiotic delivery, sequential blood samples were obtained at hours 0, 1, 2, 3, and 4 hours and then every 2 hours. Serum samples were frozen.
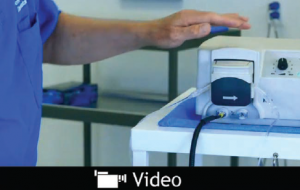
Video Graphic 2. See video, Supplemental Digital Content 11, which demonstrates the technique for painless subcutaneous infiltration of large volumes of TlA using multiholed stainless steel cannulas. This video is available in the related Videos section of the Full-Text article on PrSGlobalOpen.com or available at http://links.lww.com/PRSGO/A444.
Sequential samples of tumescent adipose tissue (10–20mL) were obtained by hand-held syringe liposuction at time T0 immediately after TAAD and continued at hours 1, 2, 3, and 4 and then every 2 hours until the subject experienced liposuction-associated pain. Samples of fat aspirate were centrifuged, supernatant fat was discarded, and infranatant TISF was frozen. Both serum and interstitial fluid samples were assayed for antibiotic concentration by high pressure liquid chromatography.
The correlation (R2) between the antibiotic concentration (mg/L) in TAAD solution and Cmax (mg/L) in liposuctioned aspirate (TISF) was determined.
The bioavailability of a drug in a tissue was measured by calculating AUC, the area under the concentration time curve C(t) over the interval (0,T0), where t = 0 is the time immediately after drug delivery and time t = T0 is the time when the drug concentration in the targeted tissue has returned to zero.
Suppose time t = T is the time of the last measurement of the drug concentration in the tissue, and that C(T) > 0, then time T < T0 and T0 must be estimated by visually extending the curve C(t) to the point where C(t) crosses the horizontal axis. Thus, AUC∞ = AUC(0, T) + AUC(T, T0), where AUC(0, T) was determined using the trapezoid
rule. AUC(T, T0) has the shape of a triangular of height
C(T), base length (T0-T) and area 1/2 C(T)(T0-T).
RESULTS
The research cohort consisted of 5 healthy adult female volunteers, ages 37–64 years. Each subject participated in 2–3 pharmacokinetic procedures. There were 15 pharmacokinetic procedures: 6 IVAD procedures and 9 TAAD procedures.
The maximum concentrations of cefazolin and metronidazole in a TAAD solution were 900mg/L and 413mg/L, respectively. See Figures 1–5 and Tables 1–5 for individual concentration–time profles. Lidocaine dosages ranged from 20 to 45mg/kg (see additional fgures and supplemental raw data, Supplemental Digital Content 1–9, http://links.lww.com/PRSGO/A442).
In TISF, peak antibiotic concentrations (Cmax) occurred at the time of the initial sample, thereafter concentrations declined linearly. TAAD produced a Cmax for both cefazolin and metronidazole in TISF approximately equal to the mg/L concentration in TAAD solution (R2 = 0.97; Fig. 6). In serum after TAAD, antibiotic Cmax occurred after 8–12 hours, thereafter concentrations declined slowly over many hours. In serum after IVAD, Cmax occurred at the time of the initial serum sample, thereafter concentrations declined exponentially.
When antibiotic doses by TAAD and IVAD were equal, the antibiotic concentrations in TISF after TAAD were strictly greater than in serum after IVAD at every time point. It follows that AUC∞, Cmax, and T > MIC (for any hypothetical MIC) in TISF after TAAD were greater than in serum after IVAD.
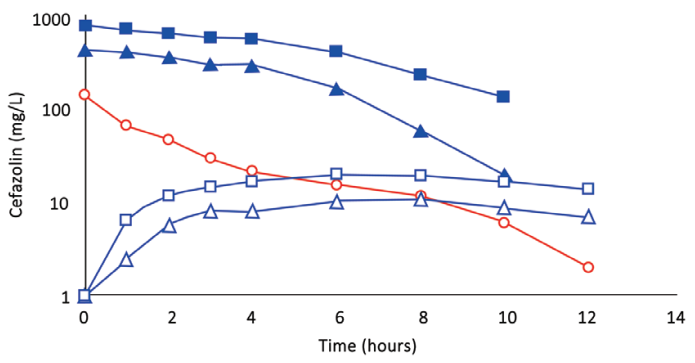
Fig. 1. (Subject 1): Comparison of concentration–time profles of cefazolin by IVAD (red) and TAAD (blue) into subcutaneous abdominal fat of a 74.3-kg female. Square symbols represent 1,000 mg by TAAD1 in a 900 mg/l solution. Triangle symbols represent 1,000 mg by TAAD2 in a 450 mg/l solution. round red symbols show 1,000 mg by IVAD. Closed symbols represent concentrations in TISF, and open symbols are concentrations in serum.
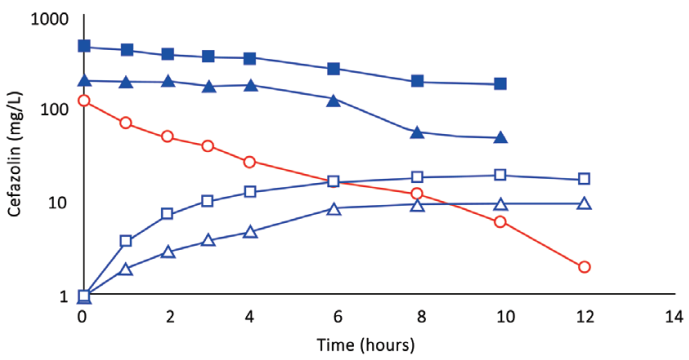
Fig. 2. (Subject 2): Comparison of concentration–time profles of cefazolin by IVAD (red) and TAAD (blue) in the breasts of a 76.4-kg female. Square symbols represent 500 mg by TAAD1 in a 450 mg/l solution (one breast). Triangle symbols represent 500 mg by TAAD2 in a 225 mg/l solution (bilateral breasts). round red symbols show 1,000 mg by IVAD. Closed symbols represent concentrations in TISF, and open symbols are concentrations in serum.
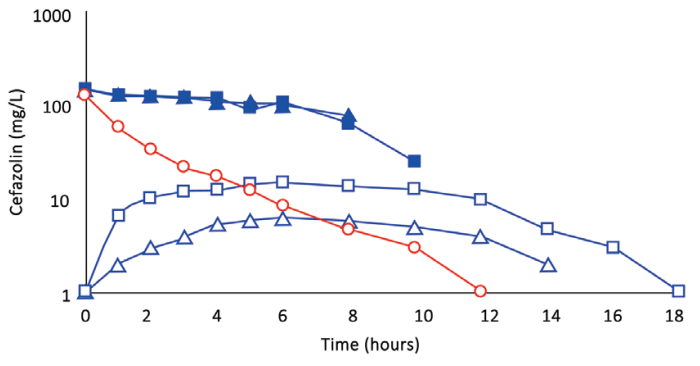
Fig. 3 (Subject 3): Comparison of concentration–time profles of cefazolin by IVAD(red) and TAAD (blue) into the hips and outer thighs of a 66.4-kg female. Square symbols represent 870 mg by TAAD1 in a 228 mg/l solution (bilateral). Triangle symbols represent 435 mg by TAAD2 in a 228 mg/l solution (one side). round symbols show 1,000 mg by IVAD. Closed symbols represent concentrations in TISF, and open symbols are concentrations in serum.
Subject 1 received 1g of cefazolin by TAAD and 1g of cefazolin by IVAD. The AUC∞ and Cmax in interstitial fluid were 16.5 and 5.6 times greater, respectively, after TAAD than in serum after IVAD.
Subject 4 received 500mg of metronidazole by TAAD and 500mg metronidazole by IVAD. The AUC∞ and Cmax for metronidazole were 8.1 and 24.7 times greater, respectively, in TISF after TAAD than in serum after IVAD.
After TAAD, the AUC∞ for cefazolin in TISF increased when the mg/L concentration of cefazolin was increased in the TAAD solution (subject 2) and when mg dose of cefazolin was increased in the TAAD solution (subject 3).
As expected, because of differences in volume of distribution and rates of clearance, equal mg doses of cefazolin and metronidazole by IVAD produced considerably different concentration–time profles in serum. In contrast, TAAD of equal mg doses and equal mg/L concentrations of cefazolin and metronidazole in the TAAD solution resulted in identical concentration–time profles in TISF (subject 4; Fig. 7).
For subject 5, when the total doses of cefazolin and metronidazole by IVAD + TAAD equaled the doses by IVAD, IVAD + TAAD resulted in more prolonged serum antibiotic concentration and higher TISF concentrations compared with IVAD alone.
The maximal rates of subcutaneous tumescent infltration ranged from 125mL/min to 250mL/min. At this rate the infltration was virtually painless.
No adverse or unusual systemic or cutaneous reactions (inflammation, tenderness, unusual ecchymosis) were observed following TAAD.
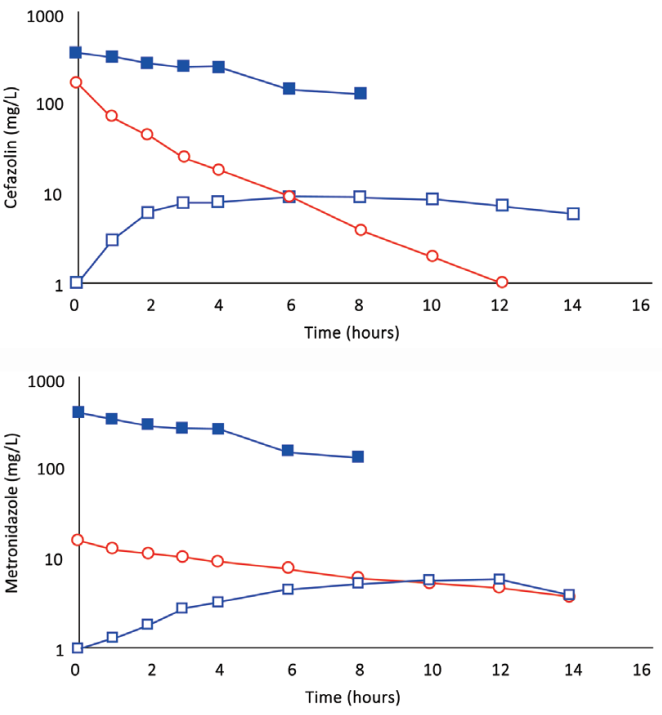
Fig. 4. A, (Subject 4): Comparison of cefazolin concentration–time profles after 500 mg by IVAD (red, round symbols) and 500 mg by TAAD (blue, square symbols) in 413 mg/l solutions into subcutaneous abdominal fat of a 66.3-kg female. Closed symbols represent concentrations in TISF, and open symbols are concentrations in serum. B (Subject 4): Comparison of metronidazole concentration–time profles after 500 mg by IVAD (red, round symbols) and 500 mg by TAAD (blue, square symbols) in 413 mg/l solutions into subcutaneous abdominal fat of a 66.3-kg female. Closed symbols represent concentrations in TISF, and open symbols are concentrations in serum.
DISCUSSION
For tumescent lidocaine at 28mg/kg in healthy subjects, the risk of mild lidocaine toxicity is 1/5,000,000.15 In patients with signifcant cardiac, hepatic, or renal impairment, the tumescent lidocaine dosages with TAAD may need to be reduced. Otherwise 28mg/kg is safe with or without general anesthesia. Anesthesiologists commonly provide general anesthesia together with IV lidocaine over 24 hours at total lidocaine dosages that can exceed 28mg/kg. At these dosages of IV lidocaine under general anesthesia toxicity is extremely rare.16
This research was an exploratory phase 1 clinical trial comparing 2 modes of antibiotic delivery: IVAD and TAAD, a novel mode of antibiotic delivery. We compared IVAD and TAAD with respect to bioavailability and maximum tissue antibiotic concentrations (Cmax).
TAAD consists of antibiotics dissolved in a solution of TLA. TLA solutions consist of lidocaine (< 1g/L), epinephrine (< 1mg/L), sodium bicarbonate (10 mEq/L) dissolved in 0.9% physiologic saline.
TLA is at least a 10-fold dilution of commercial 1% lidocaine with epinephrine 1:100,000. TLA provides long-lasting (≥ 8 hours) local anesthesia, local subcutaneous vasoconstriction, and profound surgical hemostasis. TLA is used for a wide range of surgical procedures.17–36
This research was intended to be a proof of concept pharmacokinetic study in anticipation of a subsequent randomized clinical trial (RCT) of TAAD + IVAD versus IVAD alone for SSI prevention. To minimize the sample size required for the RCT, the target population ought to have a high risk of SSI. The risk of SSI typically exceeds 15% among open colorectal surgeries in diabetic, obese, immunocompromised, or trauma patients. We chose to study cefazolin and metronidazole because they are generic, effective, inexpensive, widely available, and commonly used for colorectal surgery SSI prophylaxis. When mixed together, cefazolin and metronidazole are stable in solution and are effective after subcutaneous injection for prevention of SSIs.37–42 TAAD may prove useful in reconstructive surgery
where SSIs are more common than in cosmetic surgery.
Effective antibiotic prophylaxis of SSIs depends on adequate antibiotic concentrations at the surgical incision
site throughout the duration of the surgical procedure.43
The risk of implant surface bioflm formation is correlated with antibiotic concentration in periimplant tissue.44
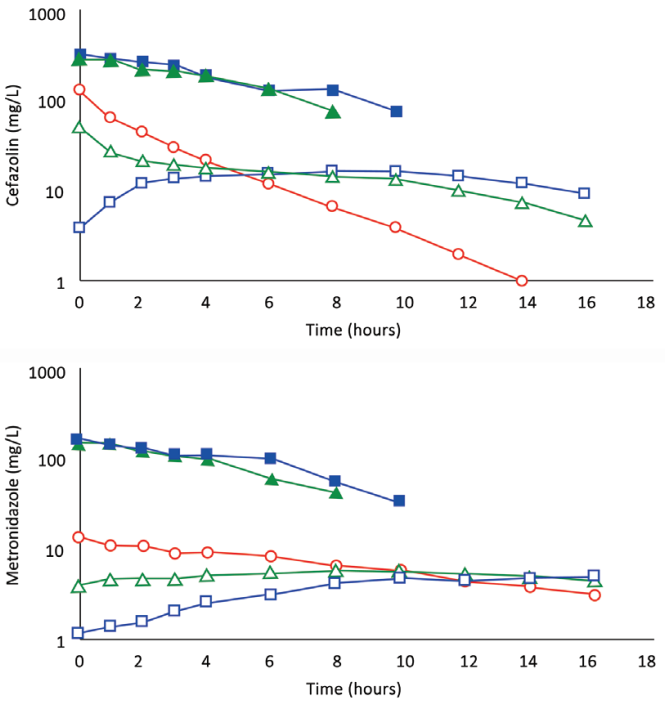
Fig. 5. A, (Subject 5): Comparison of concentration–time profles following 1,200 mg of cefazolin by IVAD1, by TAAD1, and by concomitant IVAD2 + TAAD2. Square blue symbols represent TAAD1 of a 1,200 mg/l solution. Triangle green symbols represent 800 mg by TAAD2 in a 800 mg/l solution with concomitant 400 mg by IVAD2. round red symbols show 1,200 mg by IVAD1. Closed symbols represent concentrations in TISF, and open symbols are concentrations in serum. B, (Subject 5): Comparison of concentration–time profles following 600 mg of metronidazole by IVAD1, TAAD1, and IVAD2 + TAAD2. Square blue symbols represent TAAD1 of a 600 mg/l solution. Triangle green symbols represent 400 mg by TAAD2 in a 400 mg/l solution with simultaneous 200 mg by IVAD2. round red symbols show 600 mg by IVAD1. Closed symbols represent concentrations in TISF, and open symbols are concentrations in serum.
The antibiotic concentration in TISF immediately after TAAD is virtually identical to the antibiotic concentration in the TAAD solution. Clinicians can use TAAD to deliver a preselected initial subcutaneous antibiotic concentration at a proposed surgical incision site. For some antimicrobials (e.g., aminoglycosides) achieving greater
TAAD-like subcutaneous antibiotic concentrations solely by IVAD might not be possible or might pose a signifcant risk of harm to patients.
At equal antibiotic doses, TAAD provides superior subcutaneous antibiotic bioavailability (AUC∞), Cmax, and T > MIC compared with IVAD. Assuming equal IV doses, the concomitant delivery by TAAD + IVAD is pharmacokinetically superior to IVAD alone.
Tumescent drug delivery has 3 distinct therapeutic properties: local physical effects, local pharmacologic effects, and systemic pharmacologic effects.
Local physical effects of TAAD include mechanical compression of capillaries and veins.
The 0.9% saline component of a tumescent solution provides prolonged local tissue hydration that prevents desiccation of the surgical wound surface. Tumescent tissue acts as a subcutaneous reservoir of 0.9% saline solution at the infltration (incision) site and can reduce IV fluid requirements.
Local pharmacologic effects of TAAD include epinephrine-induced prolonged and profound capillary vasoconstriction. TAAD takes advantage of the fact that subcutaneous drug absorption is perfusion-rate limited. Reduced subcutaneous blood flow with TAAD effectively isolates the TISF from the systemic circulation resulting in sustained local drug action. The concentration of a TAAD solution of antibiotics that is nontoxic to subcutaneous tissues may far exceed the antibiotic concentration that can be reliably achieved in subcutaneous tissue by IVAD.
Continuous IV infusion of antibiotics may be more effective than intermittent bolus IV infusion in acutely ill
patients.44 Continuous IV infusion may reduce the emergence of bacterial resistance and overcome existing resistant bacteria.45,46 Slow systemic absorption of lidocaine and antibiotics following TAAD produces serum concentration–time profles that resemble a slow constant IV infusion persisting for 12 hours or more.
TAAD maximizes peak drug concentrations in subcutaneous tissue while simultaneously minimizing serum Cmax,
compared with IVAD. For surgical procedures confned to subcutaneous tissue, TAAD minimizes the peak antibiotic
concentration to which gut microflora are exposed.
Following IVAD, concentrations of cefazolin and metronidazole are typically lower in interstitial fluid than in serum.48–50 Our results show that concentrations in serum after IVAD were always less than in TISF after TAAD, at every time point. Thus, TAAD + IVAD is always superior to IVAD alone with respect to AUC∞, Cmax, and T>MIC in subcutaneous interstitial fluid and in serum, assuming equal IVAD doses. This suggests that TAAD + IVAD ought to improve antibiotic prophylaxis of wound infections.
TAAD + IVAD may be superior for preventing the emergence of bacterial antibiotic resistance. The emergence of bacterial antibiotic resistance is concentration dependent. Resistant mutants proliferate at antibiotic concentrations between the MIC and the (higher) mutant prevention concentration (MPC) but do not proliferate at concentrations below the MIC or above the MPC.51
Antibiotic delivery to a surgical incision site is reduced as a result of decreased local blood flow due to wound
cautery, capillary thrombosis, and tissue desiccation. After IVAD, a concentration gradient can develop between an incision site surface (lower concentration) and the vascular compartment (higher concentration).
This concentration gradient may allow resistant mutants at an incision site to evade competition and flourish by invading tissues with higher drug concentrations, where less resistant strains do not survive.52,53 The TAAD concentrations above MPC at a surgical incision site, the site of potential bacterial contamination, may improve SSI prophylaxis and bacterial resistance prevention.54,55
TAAD alone is pharmacokinetically superior to IVAD for achieving high, prolonged subcutaneous antibiotic concentrations in wounds confned to skin and subcutaneous tissue. This suggests the hypothesis that TAAD alone may reduce the risk of incision site wound infection and bioflm formation of subcutaneous implanted devices.
TAAD + IVAD is pharmacokinetically superior to TAAD alone or IVAD alone, assuming equal IVAD doses, for achieving high, prolonged subcutaneous and systemic antibiotic concentrations. This suggests a second hypothesis that TAAD + IVAD is superior to IVAD alone for preventing SSI in wounds involving deep organs and tissues.
Cefazolin and metronidazole have distinctly different pharmacokinetic characteristics after IVAD. But after TAAD, the subcutaneous concentration–time profles of cefazolin and metronidazole are virtually identical. This suggests that diverse drugs, including antibiotic, antiviral, antifungal, and antitumor drugs, may have similar subcutaneous concentration–time profles in TISF after tumescent delivery.
We found no adverse effects of tumescent cefazolin, metronidazole, lidocaine, or epinephrine. The subcutaneous injection of cefazolin and metronidazole is “off-label” according to United States Food and Drug Administration–approved package insert labeling. Yet, subcutaneous antibiotic delivery for systemic effect is commonly used for palliative therapy.56–65 These reports and our present data suggest that tumescent delivery of dilute cefazolin and metronidazole represents a nonsignifcant risk of harm to patients.
Prolonged open gastrointestinal surgical procedures exceeding 4 hours require redosing with IV antibiotics for optimal SSI prophylaxis.66 There is a high rate of noncompliance with intraoperative redosing.67 Our data suggests that the combination of TAAD + IVAD provides prolonged high antibiotic concentrations in TISF and serum and may reduce the risk of SSI associated with noncompliance to redosing requirements.68
CLINICAL APPLICATION OF TAAD
In clinical practice, we suggest using 1L or more of TAAD solution consisting of 1g cefazolin, 500mg of metronidazole, 1g of lidocaine with 1mg epinephrine, and 10 mEq sodium bicarbonate per 1L bag of 0.9% physiologic saline or Ringer’s lactate.
Stainless steel tumescent infltration cannulas or plastic tumescent subcutaneous catheters are used for effcient, painless TAAD (Fig. 8, 9).
A trained nurse can perform TAAD infiltration in the patient’s hospital room or in the preoperative area 1–3 hours before incision, thus reducing the surgeon’s work load in the operating room and allowing time for detumescence and optimal interstitial distribution of the TAAD solution.
The prolonged high tumescent antibiotics concentrations in subcutaneous tissue may reduce the need to precisely give IV antibiotics 30–60 minutes before an incision.
With detumescence, tissue remains anesthetic and vasoconstricted for hours while becoming less waterlogged
and more easily manipulated.
THERAPEUTIC PROPERTIES OF TUMESCENT LIDOCAINE
The 0.09% lidocaine in the TAAD solution reduces the pain associated with antibiotic injection, provides immediate onset of local surgical anesthesia and prolonged postoperative analgesia. The lidocaine component of a TAAD solution has signifcant antibacterial, antiplatelet, and systemic antiinflammatory effects.
Lidocaine in vivo is bactericidal at 3mM (0.09%), the concentration of lidocaine in a TAAD solution.69–72 The lidocaine in a TAAD solution may act synergistically with TAAD antibiotics to kill bacteria. Antibacterial effects of lidocaine have been attributed to disruption of microbial cell membrane permeability by transmembrane anion (Na+, K+, Ca++) channel blockade.73–75 Slow constant systemic absorption of lidocaine prevents SSI in a mouse model.70
Lidocaine impairs platelet function at tumescent lidocaine concentrations (≥ 3mM) and may reduce the risk
of venous thromboembolism.6–80 Although tumescent lidocaine impairs platelet activation, it does not impair
surgical hemostasis.81
Lidocaine has signifcant pharmacologic antiinflammatory properties at clinically safe serum concentrations. Lidocaine may inhibit systemic inflammatory responses to surgical trauma and bacterial infection.81–96 Tumescent infltration into traumatized or infected tissue engulfs large volumes of damaged tissue, which may prevent or delay the systemic absorption of inflammatory cytokines, chemokines, histones, and pathogens.
The continuous systemic absorption of up to 28mg/kg of tumescent lidocaine provides predictably safe convenient therapeutic serum lidocaine concentrations, in the range of 1–3mg/L, for up to 24 hours or more. Systemic lidocaine has been reported to provide preemptive, interoperative, and postoperative analgesia.97 Lidocaine local anesthesia reduces postoperative narcotic use with earlier return of normal bowl function and earlier postoperative ambulation.98–101 Sodium bicarbonate in the TAAD solution raises the pH of the solution and eliminates the stinging pain associated with acidic pH of commercial solutions of lidocaine and epinephrine.102
RISKS OF TUMESCENT DRUG DELIVERY
Human error is the most dangerous aspect of tumescent drug delivery. To avoid miscommunication, legible written
physician orders must specify the amount of lidocaine in terms of total milligrams per bag of tumescent solution and
total mg/kg dosage permitted for any individual patient. Every bag of TAAD solution must be clearly labeled on both sides with tumescent safety labels that state, “Subcutaneous Tumescent Lidocaine, Not for IV.” Inadvertent IV delivery of a TAAD solution must be avoided.
LIMITATIONS OF THIS STUDY
This research has involved a limited number of patients and is not designed to replicate procedures, test hypotheses, or estimate pharmacokinetic parameters. Our data suggest that TAAD + IVAD is pharmacokinetically superior to IVAD for SSI prevention, but it does not show that TAAD + IVAD is clinically superior to IVAD for SSI prevention.
Some measurements of antibiotic tissue concentrations were discontinued before complete clearance of the antibiotic. In such cases, the last measured antibiotic concentration was > 0, and the estimation of AUC∞ required a subjective visual estimation of the terminal concentration–time curve. Nevertheless, the differences between clinically measured portions of the AUC∞ were so pronounced that the size of the last portion of the AUC∞ did not affect the conclusions.
It is plausible that the transient tumescent vasoconstriction and local tissue hypoxia produced by TLA may adversely affect the incidence of SSIs. However, the incidence of SSIs associated with large volume tumescent liposuction totally by local anesthesia performed under standard aseptic technique is extraordinarily small.103–106
We only investigated the use of cefazolin and metronidazole. The use of other antibiotics for TAAD or IVAD
requires clinical investigation to document its safety.
FUTURE RESEARCH
We propose a multicenter RCT of concomitant TAAD + IVAD versus IVAD alone, with the primary endpoint being a reduced incidence of SSI for a variety of surgical procedures. Secondary endpoints are postoperative thromboembolism, surgical blood loss, systemic and local inflammatory response to surgical trauma and surgery, postoperative narcotic requirements, time to postoperative ambulation, and length of stay in hospital. Potential target population include patients undergoing procedures at high risk for SSIs, such those involving prolonged complex plastic surgical procedures, contaminated abdominal wounds, diabetes, impaired immunity, combat wounds, burns, and patients within medically indigent communities (Supplemental Digital Content 2, http://links.lww.com/PRSGO/A442)
CONCLUSIONS
Subcutaneous antibiotic bioavailability using TAAD is superior to that of IVAD. The hypothesis that combined TAAD + IVAD is more effective than IVAD alone for SSI prevention, assuming equal IV antibiotic doses, remains to be tested with RCT. There was no evidence that TAAD of cefazolin and metronidazole poses a signifcant risk of harm to patients.
Department of Dermatology
School of Medicine, University of California, Irvine
30280 Rancho Viejo Road
San Juan Capistrano, CA 92675
E-mail: jeff@kleinmd.com
ACKNOWLEDGMENTS
Atoussa Cameron, RN, NP, and JoAnn Coker, RN, NP, assisted in caring for patients and obtaining the serum samples. Jonathan Good, Toxicology/Drug Monitoring Lab, Mayo Clinic, Rochester, Minn., performed the cefazolin high-performance liquid chromatography assays. Paytra Klein proof read drafts of the article and checked the accuracy of reference citations. Susan Ruffalo edited an early version of the article.
DISCLOSURES
*Dr. Klein owns patents on devices for tumescent drug delivery by subcutaneous infltration. Dr. Klein’s wife, Kathleen Hutton-Klein, MD, owns HK Surgical, Inc., a company that sells devices for tumescent drug delivery.
†Dr. Langman has no conflicts of interest to report. This was investigator-initiated research, funded entirely by the authors.
The Article Processing Charge was paid for by the authors.
REFERENCES
1. Boehm O, Baumgarten G, Hoeft A. Epidemiology of the highrisk population: perioperative risk and mortality after surgery.
Curr Opin Crit Care. 2015;21:322–327.
2. Aiken AM, Karuri DM, Wanyoro AK, et al. Interventional studies
for preventing surgical site infections in sub-Saharan Africa—a
systematic review. Int J Surg. 2012;10:242–249.
3. Viet Hung N, Anh Thu T, Rosenthal VD, et al. Surgical site infection rates in seven cities in Vietnam: fndings of the International
Nosocomial Infection Control Consortium. Surg Infect (Larchmt).
2016;17:243–249.
4. Toma O, Suntrup P, Stefanescu A, et al. Pharmacokinetics and
tissue penetration of cefoxitin in obesity: implications for risk of
surgical site infection. Anesth Analg. 2011;113:730–737.
5. Hu H, Johani K, Almatroudi A, et al. Bacterial bioflm infection
detected in breast implant-associated anaplastic large-cell lymphoma. Plast Reconstr Surg. 2016;137:1659–1669.
6. Deva AK, Adams WP, Jr, Vickery K. The role of bacterial bioflms in
device-associated infection. Plast Reconstr Surg. 2013;132:1319–1328.
7. Giormezis N, Kolonitsiou F, Foka A, et al. Coagulase-negative
staphylococcal bloodstream and prosthetic-device-associated infections: the role of bioflm formation and distribution of adhesin and toxin genes. J Med Microbiol. 2014;63:1500–1508.
8. Franchelli S, Pesce M, Savaia S, et al. Clinical and microbiological characterization of late breast implant infections after reconstructive breast cancer surgery. Surg Infect (Larchmt).
2015;16:636–644.
9. Bhargava P, Mehrotra N, Kumar A. Wound infection after metronidazole infltration. Trop Doct. 2006;36:37–38.
10. Shubing W, Litian Z. Preventing infection of the incision after
appendectomy by using metronidazole preoperatively to infltrate tissues at the incision. Am J Surg. 1997;174:422–424.
11. Quendt J, Blank I, Seidel W. [Peritoneal and subcutaneous administration of cefazolin as perioperative antibiotic prophylaxis
in colorectal operations. Prospective randomized comparative
study of 200 patients]. Langenbecks Arch Chir. 1996;381:318–322.
12. el-Sef TA, el-Awady HM, Shehata MI, et al. Systemic plus local
metronidazole and cephazolin in complicated appendicitis: a
prospective controlled trial. J R Coll Surg Edinb. 1989;34:13–16.
13. Dixon JM, Armstrong CP, Duffy SW, et al. A randomized prospective trial comparing the value of intravenous and preincisional cefamandole in reducing postoperative sepsis after operations upon
the gastrointestinal tract. Surg Gynecol Obstet. 1984;158:303–307.
14. Pollock AV, Evans M, Smith GM. Preincisional intraparietal
Augmentin in abdominal operations. Ann R Coll Surg Engl.
1989;71:97–100.
15. Klein JA, Jeske DR. Estimated maximal safe dosages of tumescent
lidocaine. Anesth Analg. 2016;122:1350–1359.
16. Dunn LK, Durieux ME. Perioperative Use of Intravenous
Lidocaine. Anesthesiology. 2017;126:729–737.
17. Klein JA. The tumescent technique for liposuction surgery. J Am
Acad Cosmetic Surg. 1987;4:263–267.
18. Shimizu Y, Nagasao T, Taneda H, et al. Combined usage of intercostal nerve block and tumescent anaesthesia: an effective anaesthesia technique for breast augmentation. J Plast Surg Hand Surg.
2014;48:51–55.
19. Rusciani A, Pietramaggiori G, Troccola A, et al. The outcome
of primary subglandular breast augmentation using tumescent
local anesthesia. Ann Plast Surg. 2016;76:13–17.
20. Sleth JC, Servais R, Saizy C. [Tumescent infltrative anaesthesia for mastectomy: about six cases]. Ann Fr Anesth Reanim.
2008;27:941–944.
21. Orgill DP. Excision and skin grafting of thermal burns. N Engl J
Med. 2009;360:893–901.
22. Gümüş N. Tumescent infltration of lidocaine and adrenaline for
burn surgery. Ann Burns Fire Disasters. 2011;24:144–148.
23. Blome-Eberwein S, Abboud M, Lozano DD, et al. Effect of subcutaneous epinephrine/saline/local anesthetic versus saline-only
injection on split-thickness skin graft donor site perfusion, healing, and pain. J Burn Care Res. 2013;34:e80–e86.
24. Cohn MS, Seiger E, Goldman S. Ambulatory phlebectomy using the tumescent technique for local anesthesia. Dermatol Surg.
1995;21:315–318.
25. Vuylsteke ME, Mordon SR. Endovenous laser ablation: a review
of mechanisms of action. Ann Vasc Surg. 2012;26:424–433.
26. Haines WY, Deets R, Lu N, et al. Tumescent anesthesia reduces
pain associated with balloon angioplasty of hemodialysis fstulas.
J Vasc Surg. 2012;56:1453–1456.
27. Behroozan DS, Goldberg LH. Dermal tumescent local anesthesia in cutaneous surgery. J Am Acad Dermatol. 2005;53:828–830.
28. Girard C, Debu A, Bessis D, et al. Treatment of Gorlin syndrome
(nevoid basal cell carcinoma syndrome) with methylaminolevulinate photodynamic therapy in seven patients, including two
children: interest of tumescent anesthesia for pain control in
children. J Eur Acad Dermatol Venereol. 2013;27:e171–e175.
29. Ramon Y, Barak Y, Ullmann Y, et al. Pharmacokinetics of highdose diluted lidocaine in local anesthesia for facelift procedures.
Ther Drug Monit. 2007;29:644–647.
30. Abramson DL. Tumescent abdominoplasty: an ambulatory offce
procedure. Aesthetic Plast Surg. 1998;22:404–407.
31. Narita M, Sakano S, Okamoto S, et al. Tumescent local anesthesia in inguinal herniorrhaphy with a PROLENE hernia system:
original technique and results. Am J Surg. 2009;198:e27–e31.
32. Locke M, Windsor J, Dunbar PR. Human adipose-derived stem
cells: isolation, characterization and applications in surgery. ANZ
J Surg. 2009;79:235–244.
33. Mizukami T, Hamamoto M. Tumescent local anesthesia for a
revascularization of a coronary subclavian steal syndrome. Ann
Thorac Cardiovasc Surg. 2007;13:352–354.
34. Prasetyono TO, Koswara AF. Linear hand burn contracture release under local anesthesia without tourniquet. Hand Surg.
2015;20:484–487.
35. Bashir MM, Qayyum R, Saleem MH, et al. Effect of time interval between tumescent local anesthesia infltration and start of
surgery on operative feld visibility in hand surgery without tourniquet. J Hand Surg Am. 2015;40:1606–1609.
36. Lalonde D, Martin A. Tumescent local anesthesia for hand surgery: improved results, cost effectiveness, and wide-awake patient
satisfaction. Arch Plast Surg. 2014;41:312–316.
37. Rivers TE, McBride HA, Trang JM. Stability of cefazolin sodium
and metronidazole at 8degrees C for use as an i.v. admixture. J
Parenter Sci Technol. 1993;47:135–137.
38. Meyer NL, Hosier KV, Scott K, et al. Cefazolin versus cefazolin
plus metronidazole for antibiotic prophylaxis at cesarean section. South Med J. 2003;96:992–995.
39. Morris WT, Innes DB, Richardson RA, et al. The prevention of
post-appendicectomy sepsis by metronidazole and cefazolin: a
controlled double blind trial. Aust N Z J Surg. 1980;50:429–433.
40. Brown GR, Clarke AM. Therapeutic interchange of cefazolin
with metronidazole for cefoxitin. Am J Hosp Pharm. 1992;49:
1946–1950.
41. Hospenthal DR, Murray CK, Andersen RC, et al.; Infectious
Diseases Society of America; Surgical Infection Society.
Guidelines for the prevention of infections associated with
combat-related injuries: 2011 update: endorsed by the Infectious
Diseases Society of America and the Surgical Infection Society. J
Trauma. 2011;71:S210–S234.
42. Cho MJ, Kurtz RR, Lewis C, et al. Metronidazole phosphate—a
water-soluble prodrug for parenteral solutions of metronidazole.
J Pharm Sci. 1982;71:410–414.
43. Mangram AJ, Horan TC, Pearson ML, et al. Guideline for prevention of surgical site infection, 1999. Centers for Disease Control
and Prevention (CDC) Hospital Infection Control Practices
Advisory Committee. Am J Infect Control. 1999;27:97–132; quiz 133.
44. Bergamini TM, Peyton JC, Cheadle WG. Prophylactic antibiotics prevent bacterial bioflm graft infection. J Surg Res. 1992;52:101–105.
45. Abdul-Aziz MH, Sulaiman H, Mat-Nor MB, et al. Beta-Lactam
Infusion in Severe Sepsis (BLISS): a prospective, two-centre,
open-labelled randomised controlled trial of continuous versus
intermittent beta-lactam infusion in critically ill patients with severe sepsis. Intensive Care Med. 2016;42:1535–1545.
46. De Waele JJ, Lipman J, Akova M, et al. Risk factors for target nonattainment during empirical treatment with β-lactam antibiotics
in critically ill patients. Intensive Care Med. 2014;40:1340–1351.
47. Abdul-Aziz MH, Lipman J, Mouton JW, et al. Applying pharmacokinetic/pharmacodynamic principles in critically ill patients:
optimizing effcacy and reducing resistance development. Semin
Respir Crit Care Med. 2015;36:136–153.
48. Brill MJ, Houwink AP, Schmidt S, et al. Reduced subcutaneous tissue distribution of cefazolin in morbidly obese versus
non-obese patients determined using clinical microdialysis. J
Antimicrob Chemother. 2014;69:715–723.
49. Hoffstedt B, Walder M. Influence of serum protein binding and
mode of administration on penetration of fve cephalosporins
into subcutaneous tissue fluid in humans. Antimicrob Agents
Chemother. 1981;20:783–786.
50. Badia JM, de la Torre R, Farré M, et al. Inadequate levels of metronidazole in subcutaneous fat after standard prophylaxis. Br J
Surg. 1995;82:479–482.
51. Strukova EN, Portnoy YA, Zinner SH, et al. Predictors of bacterial resistance using in vitro dynamic models: area under the
concentration-time curve related to either the minimum inhibitory or mutant prevention antibiotic concentration. J Antimicrob
Chemother. 2016;71:678–684.
52. Hermsen R, Deris JB, Hwa T. On the rapidity of antibiotic resistance evolution facilitated by a concentration gradient. Proc Natl
Acad Sci U S A. 2012;109:10775–10780.
53. Hol FJ, Hubert B, Dekker C, et al. Density-dependent adaptive
resistance allows swimming bacteria to colonize an antibiotic gradient. ISME J. 2016;10:30–38.
54. Zelenitsky SA, Ariano RE, Harding GK, et al. Antibiotic pharmacodynamics in surgical prophylaxis: an association between
intraoperative antibiotic concentrations and effcacy. Antimicrob
Agents Chemother. 2002;46:3026–3030.
55. Nielsen EI, Friberg LE. Pharmacokinetic-pharmacodynamic modeling of antibacterial drugs. Pharmacol Rev. 2013;65:1053–1090.
56. Azevedo EF, Barbosa LA, DeBortoli Cassiani SH. Administration
of antibiotics subcutaneously: an integrative literature review.
Acta Paul Enferm. 2012;25:817–22.
57. Robelet A, Caruba T, Corvol A, et al. [Antibiotics given subcutaneously to elderly]. Presse Med. 2009;38:366–376.
58. Frasca D, Marchand S, Petitpas F, et al. Pharmacokinetics of ertapenem following intravenous and subcutaneous infusions in
patients. Antimicrob Agents Chemother. 2010;54:924–926.
59. Walker P, Neuhauser MN, Tam VH, et al. Subcutaneous administration of cefepime. J Pain Symptom Manage. 2005;30:170–174.
60. Melin-Coviaux F, Hary L, Hurtel AS, et al. Etude pharmaco-clinique comparative de la ceftriaxone par voie sous-cutanee et intraveineuse chez la personne agee. Revue Geriatr. 2000;25:337–347.
61. Bricaire F, Castaing JL, Pocidalo JJ, et al. [Pharmacokinetics and
tolerance of ceftriaxone after subcutaneous administration].
Pathol Biol (Paris). 1988;36:702–705.
62. Borner K, Lode H, Hampel B, et al. Comparative pharmacokinetics of ceftriaxone after subcutaneous and intravenous administration. Chemotherapy. 1985;31:237–245.
63. Gauthier D, Schambach S, Crouzet J, et al. Subcutaneous and
intravenous ceftriaxone administration in patients more than 75
years of age. Med Mal Infect. 2014;44:275–280.
64. Barbot A, Venisse N, Rayeh F, et al. Pharmacokinetics and pharmacodynamics of sequential intravenous and subcutaneous teicoplanin in critically ill patients without vasopressors. Intensive
Care Med. 2003;29:1528–1534.
65. Champoux N, Du Souich P, Ravaoarinoro M, et al. Single-dose
pharmacokinetics of ampicillin and tobramycin administered by
hypodermoclysis in young and older healthy volunteers. Br J Clin
Pharmacol. 1996;42:325–331.
66. Jeong SJ, Ann HW, Kim JK, et al. Incidence and risk factors for
surgical site infection after gastric surgery: a multicenter prospective cohort study. Infect Chemother. 2013;45:422–430.
67. Goede WJ, Lovely JK, Thompson RL, et al. Assessment of prophylactic antibiotic use in patients with surgical site infections. Hosp
Pharm. 2013;48:560–567.
68. Adembri C, Ristori R, Chelazzi C, et al. Cefazolin bolus and continuous administration for elective cardiac surgery: improved
pharmacokinetic and pharmacodynamic parameters. J Thorac
Cardiovasc Surg. 2010;140:471–475.
69. Stratford AF, Zoutman DE, Davidson JS. Effect of lidocaine and
epinephrine on Staphylococcus aureus in a guinea pig model of
surgical wound infection. Plast Reconstr Surg. 2002;110:1275–1279.
70. Lu CW, Lin TY, Shieh JS, et al. Antimicrobial effect of continuous
lidocaine infusion in a Staphylococcus aureus-induced wound infection in a mouse model. Ann Plast Surg. 2014;73:598–601.
71. Parr AM, Zoutman DE, Davidson JS. Antimicrobial activity of
lidocaine against bacteria associated with nosocomial wound infection. Ann Plast Surg. 1999;43:239–245
72. Igarashi T, Suzuki T, Mori K, et al. The effects of epidural anesthesia on growth of Escherichia coli at pseudosurgical site: the
roles of the lipocalin-2 pathway. Anesth Analg. 2015;121:81–89.
73. Lee S, Goodchild SJ, Ahern CA. Local anesthetic inhibition of a
bacterial sodium channel. J Gen Physiol. 2012;139:507–516.
74. Rodrigues AA, Pina-Vaz C, Mårdh PA, et al. Inhibition of
germ tube formation by Candida albicans by local anesthetics: an effect related to ionic channel blockade. Curr Microbiol.
2000;40:145–148.
75. Johnson SM, Saint John BE, Dine AP. Local anesthetics as antimicrobial agents: a review. Surg Infect (Larchmt). 2008;9:205–213.
76. Nachmias V, Sullender J, Asch A. Shape and cytoplasmic flaments in control and lidocaine-treated human platelets. Blood.
1977;50:39–53.
77. Huang GS, Lin TC, Wang JY, et al. Lidocaine priming reduces
ADP-induced P-selectin expression and platelet-leukocyte aggregation. Acta Anaesthesiol Taiwan. 2009;47:56–61.
78. Tobias MD, Pilla MA, Rogers C, et al. Lidocaine inhibits blood
coagulation: implications for epidural blood patch. Anesth Analg.
1996;82:766–769.
79. Grant GJ, Ramanathan S, Patel N, et al. The effects of local
anesthetics on maternal and neonatal platelet function. Acta
Anaesthesiol Scand. 1989;33:409–412.
80. Pinto LM, Pereira R, de Paula E, et al. Influence of liposomal
local anesthetics on platelet aggregation in vitro. J Liposome Res.
2004;14:51–59.
81. Klein JA. Tumescent technique for local anesthesia improves safety in large-volume liposuction. Plast Reconstr Surg. 1993;92:1085–
1098; discussion 1099.
82. Hahnenkamp K, Theilmeier G, Van Aken HK, et al. The effects
of local anesthetics on perioperative coagulation, inflammation,
and microcirculation. Anesth Analg. 2002;94:1441–1447.
83. Wright JL, Durieux ME, Groves DS. A brief review of innovative uses for local anesthetics. Curr Opin Anaesthesiol. 2008;21:
651–656.
84. Hatakeyama N, Matsuda N. Alert cell strategy: mechanisms of
inflammatory response and organ protection. Curr Pharm Des.
2014;20:5766–5778.
85. Berger C, Rossaint J, Van Aken H, et al. Lidocaine reduces neutrophil recruitment by abolishing chemokine-induced arrest
and transendothelial migration in septic patients. J Immunol.
2014;192:367–376.
86. Wang HL, Liu YY, Yan HD, et al. Intraoperative systemic lidocaine inhibits the expression of HMGB1 in patients undergoing
radical hysterectomy. Int J Clin Exp Med. 2014;7:3398–3403.
87. Wang HL, Zhang WH, Lei WF, et al. The inhibitory effect
of lidocaine on the release of high mobility group box 1 in
lipopolysaccharide-stimulated macrophages. Anesth Analg.
2011;112:839–844.
88. Van Der Wal S, Vaneker M, Steegers M, et al. Lidocaine increases
the anti-inflammatory cytokine IL-10 following mechanical ventilation in healthy mice. Acta Anaesthesiol Scand. 2015;59:47–55.
89. Lee PY, Tsai PS, Huang YH, et al. Inhibition of toll-like receptor-4,
nuclear factor-kappaB and mitogen-activated protein kinase by
lignocaine may involve voltage-sensitive sodium channels. Clin
Exp Pharmacol Physiol. 2008;35:1052–1058.
90. Kiyonari Y, Nishina K, Mikawa K, et al. Lidocaine attenuates
acute lung injury induced by a combination of phospholipase A2
and trypsin. Crit Care Med. 2000;28:484–489.
91. Nishina K, Mikawa K, Takao Y, et al. Intravenous lidocaine attenuates acute lung injury induced by hydrochloric acid aspiration in rabbits. Anesthesiology. 1998;88:1300–1309.
92. Benlier E, Eskiocak S, Puyan FO, et al. Effect of lidocaine on
reducing injury in a rat electrical burn model. Ann Plast Surg.
2012;69:152–156.
93. Liu J, Zhang H, Qi Z, et al. Lidocaine protects against renal
and hepatic dysfunction in septic rats via downregulation of
Toll‑like receptor 4. Mol Med Rep. 2014;9:118–124.
94. Peiró JR, Barnabé PA, Cadioli FA, et al. Effects of lidocaine infusion during experimental endotoxemia in horses. J Vet Intern
Med. 2010;24:940–948.
95. Gallos G, Jones DR, Nasr SH, et al. Local anesthetics reduce
mortality and protect against renal and hepatic dysfunction in
murine septic peritonitis. Anesthesiology. 2004;101:902–911.
96. Schmidt W, Schmidt H, Bauer H, et al. Influence of lidocaine
on endotoxin-induced leukocyte-endothelial cell adhesion and
macromolecular leakage in vivo. Anesthesiology. 1997;87:617–624.
97. De Oliveira GS, Jr, Fitzgerald P, Streicher LF, et al. Systemic lidocaine to improve postoperative quality of recovery after ambulatory laparoscopic surgery. Anesth Analg. 2012;115:262–267.
98. Fierheller EE, Caulkett NA, Haley DB, et al. Onset, duration
and effcacy of four methods of local anesthesia of the horn
bud in calves. Vet Anaesth Analg. 2012;39:431–435.
99. Katz J, Clarke H, Seltzer Z. Review article: preventive analgesia:
quo vadimus? Anesth Analg. 2011;113:1242–1253.
100. Rosaeg OP, Bell M, Cicutti NJ, et al. Pre-incision infltration
with lidocaine reduces pain and opioid consumption after reduction mammoplasty. Reg Anesth Pain Med. 1998;23:575–579.
101. Cui W, Li Y, Li S, et al. Systemic administration of lidocaine
reduces morphine requirements and postoperative pain of patients undergoing thoracic surgery after propofol-remifentanilbased anaesthesia. Eur J Anaesthesiol. 2010;27:41–46.
102. McKay W, Morris R, Mushlin P. Sodium bicarbonate attenuates
pain on skin infltration with lidocaine, with or without epinephrine. Anesth Analg. 1987;66:572–574.
103. Coldiron BM, Healy C, Bene NI. Offce surgery incidents: what
seven years of Florida data show us. Dermatol Surg. 2008;34:285–
291; discussion 291.
104. Grazer FM, de Jong RH. Fatal outcomes from liposuction: census survey of cosmetic surgeons. Plast Reconstr Surg.
2000;105:436–446; discussion 447.
105. Lehnhardt M, Homann HH, Daigeler A, et al. Major and lethal
complications of liposuction: a review of 72 cases in Germany
between 1998 and 2002. Plast Reconstr Surg. 2008;121:396e–
403e.
106. Habbema L. Safety of liposuction using exclusively tumescent local anesthesia in 3,240 consecutive cases. Dermatol Surg.
2009;35:1728–1735.